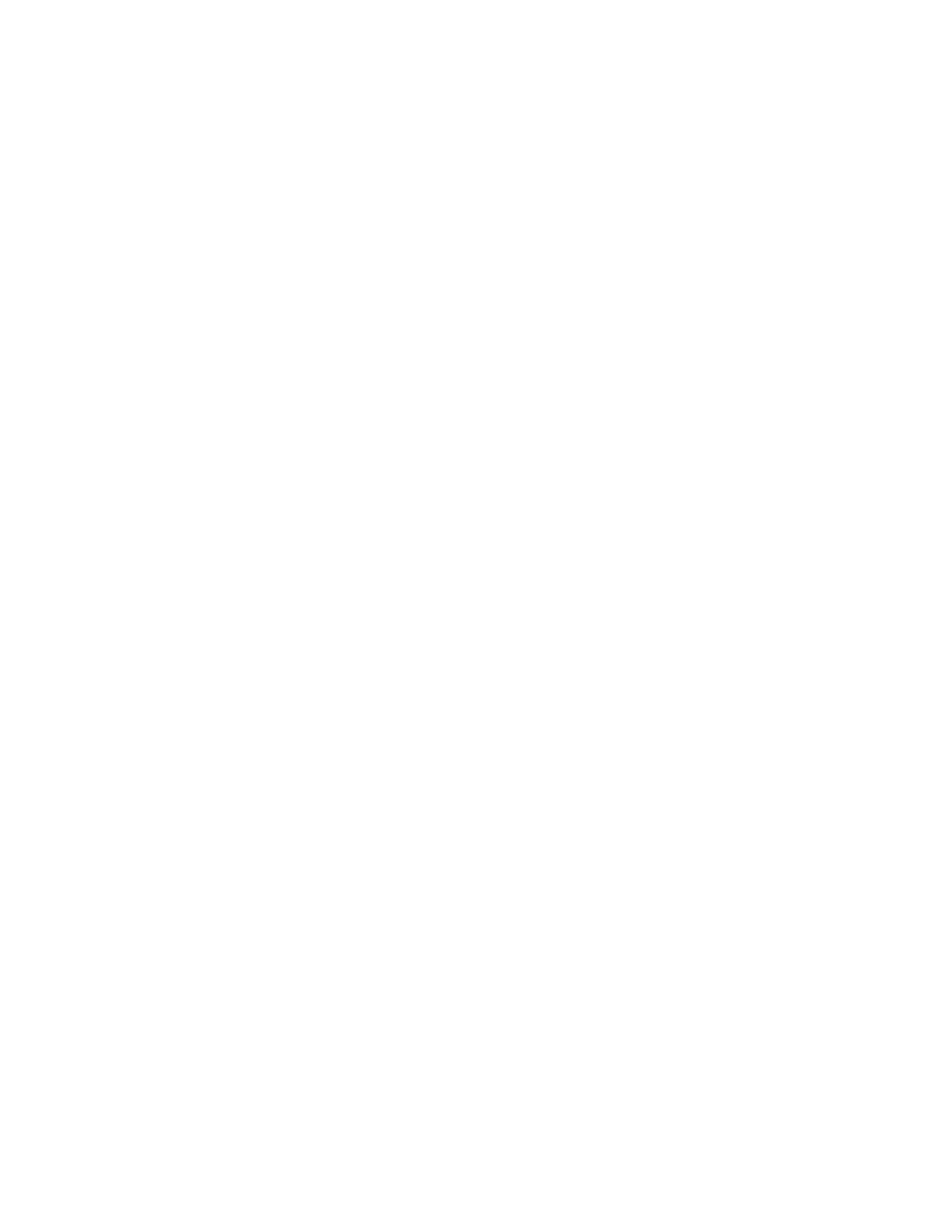
Manual: 151-082010 300-302 Series Page 3 of 31
Table of Contents
1. GENERAL INFORMATION............................................................................................................................................4
1.1. FEATURES....................................................................................................................................................................4
1.2. SPECIFICATIONS...........................................................................................................................................................5
1.3. OPTIONAL 4-20 MA CURRENT OUTPUT............................................................................................................................ 5
1.4. OTHER ACCESSORIES ......................................................................................................................................................6
1.4.1. Hastings Power supplies........................................................................................................................................6
1.4.2. Interconnecting Cables...........................................................................................................................................6
2. INSTALLATION AND OPERATION.............................................................................................................................7
2.1. RECEIVING INSPECTION ............................................................................................................................................... 7
2.2. POWER REQUIREMENTS ...............................................................................................................................................7
2.3. OUTPUT SIGNAL...........................................................................................................................................................7
2.4. MECHANICAL CONNECTIONS.......................................................................................................................................8
2.4.1. Filtering..................................................................................................................................................................8
2.4.2. Mounting ................................................................................................................................................................8
2.4.3. Plumbing ................................................................................................................................................................8
2.5. ELECTRICAL CONNECTIONS.........................................................................................................................................8
2.6. OPERATION..................................................................................................................................................................9
2.6.1. Operating Conditions.............................................................................................................................................9
2.6.2. Zero Check...........................................................................................................................................................10
2.6.3. High Pressure Operation .....................................................................................................................................10
2.6.4. Blending of Gases................................................................................................................................................. 11
2.7. OUTPUT FILTER .........................................................................................................................................................12
2.8. CONTROLLING OTHER PROCESS VARIABLES .............................................................................................................12
2.9. COMMAND INPUT.......................................................................................................................................................13
2.10. VALVE-OVERRIDE CONTROL.....................................................................................................................................13
2.11. GAIN POTENTIOMETER ..............................................................................................................................................13
2.12. TEMPERATURE COEFFICIENTS ...................................................................................................................................14
3. THEORY OF OPERATION...........................................................................................................................................15
3.1. OVERALL FUNCTIONAL DESCRIPTION........................................................................................................................15
3.2. SENSOR DESCRIPTION................................................................................................................................................ 15
3.3. SENSOR THEORY........................................................................................................................................................15
3.4. BASE.......................................................................................................................................................................... 17
3.5. SHUNT DESCRIPTION..................................................................................................................................................17
3.6. SHUNT THEORY .........................................................................................................................................................17
3.7. CONTROL VALVE.......................................................................................................................................................21
3.8. ELECTRONIC CIRCUITRY............................................................................................................................................21
4. MAINTENANCE..............................................................................................................................................................22
4.1. TROUBLESHOOTING ...................................................................................................................................................22
4.2. ADJUSTMENTS ........................................................................................................................................................... 23
4.2.1. Calibration Procedure ......................................................................................................................................... 23
4.3. END CAP REMOVAL...................................................................................................................................................23
4.4. PRINTED CIRCUIT BOARD REPLACEMENT..................................................................................................................24
4.5. SENSOR REPLACEMENT .............................................................................................................................................24
5. GAS CONVERSION FACTORS....................................................................................................................................25
6. VOLUMETRIC VS MASS FLOW.................................................................................................................................27
7. DRAWINGS AND REFERENCES ................................................................................................................................28
8. WARRANTY....................................................................................................................................................................31
8.1. WARRANTY REPAIR POLICY ......................................................................................................................................31
8.2. NON-WARRANTY REPAIR POLICY .............................................................................................................................31