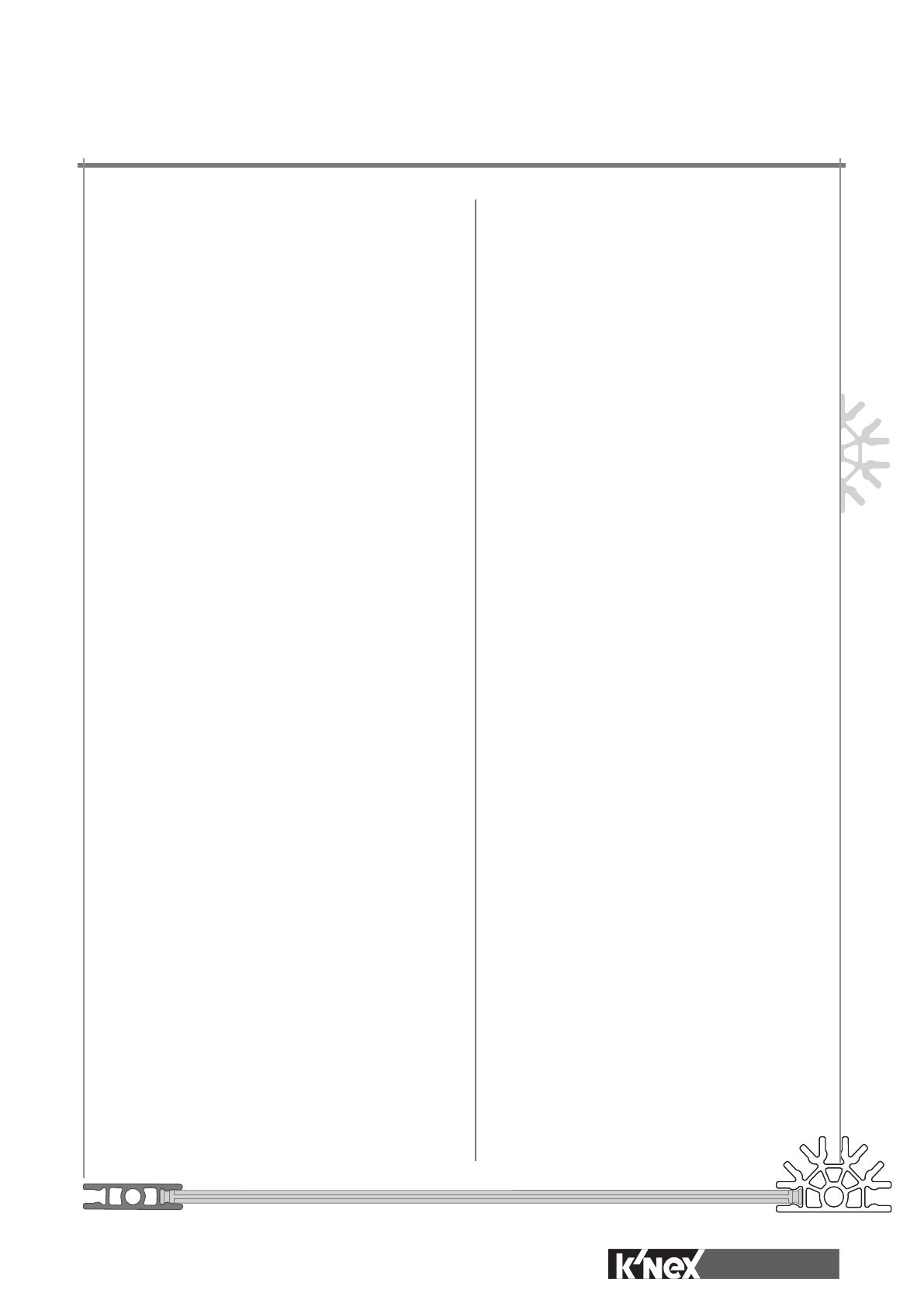
Introduction
Introduction to Structures: Bridges
• Implementingtheideasandinformation
contained in the Guide can build your
pupils’ knowledge and understanding of
structures and the forces acting on them.
• Keybackgroundinformationisprovided
in “ A Quick Start Guide” while the
Lesson Notes for the K’NEX models
provide more detailed information and
ideas for possible teaching activities.
These teaching activities have been
developed primarily to support the
DfEE/QCA Scheme of Work for Key
Stages 1 and 2 in:
•DesignandTechnologyUnit6A:
‘Shelters’ and related units
involving structures
•ScienceUnit6E:
‘Balanced and unbalanced forces’
and related units
•ICTUnit5B:
‘Analysing data and asking questions:
using complex searches’ and related
units involving research using Internet
search engines.
• Aglossaryoftechnicaltermsisofferedas
a resource for the teacher.
• Eachofthelessonscanbecompleted
in approximately 1-2 hours but can be
extended using suggested Extension and
Research Activities. Useful Internet web
sites are listed to help guide the research
activities. (Note: these were functioning
sites at the time of going to print.)
• Aselectionofworksheetsisprovidedfor
your classroom use. These can be used
for enrichment and assessment activities.
• Theteachingactivitiesareintendedto
encourage the development of key skills
by providing opportunities for whole
class and group discussions, observing,
evaluating and recording through the use
of text and drawings, working with others
to solve problems and using ICT within a
design and technology context.
Education®
Education®
This K’NEX Bridges kit is the first in a
series called Understanding Structures.
An investigation of how bridges work will
help children develop a good
understanding of the forces affect-
ing structures in general. A successful
bridge is the result of balanced forces
in action. While bridge building makes
use of simple scientific concepts, their
application often requires complex
engineering solutions. It also demands
a knowledge and understanding of
the physical properties of materials
so that their influence on the design
and construction of the bridge can
be determined.
The K’NEX Introduction to Structures:
Bridges Kit
• Developedtointroducepupilstothe
7 main types of bridge design, this
construction kit also provides opportunities
for learning how structures are made
stable and how they are able to support
their loads. In addition, it can be used to
demonstrate that structures can fail when
loaded and to identify the techniques for
reinforcing and strengthening them.
• Workinginpairsorsmallcollaborative
groups, the kit provides opportunities for
pupils to explore different bridge designs,
and the forces acting on them, through the
use of focused practical tasks (FPTs), and
investigative, disassembling and evaluative
activities (IDEAs). The information gained
from these activities will help children
develop their own ideas to solve a bridge
design problem using sheet and other
resistant materials, as well as K’NEX
construction kits, where appropriate.
Teacher Support Materials
• Developedinitiallyforthenon-specialist
teacher, the materials included in the
Teacher’s Guide can also be used as a
resource by more experienced teachers
as they develop their own lesson plans.
3
bridges
website: www.knexeducation.co.uk