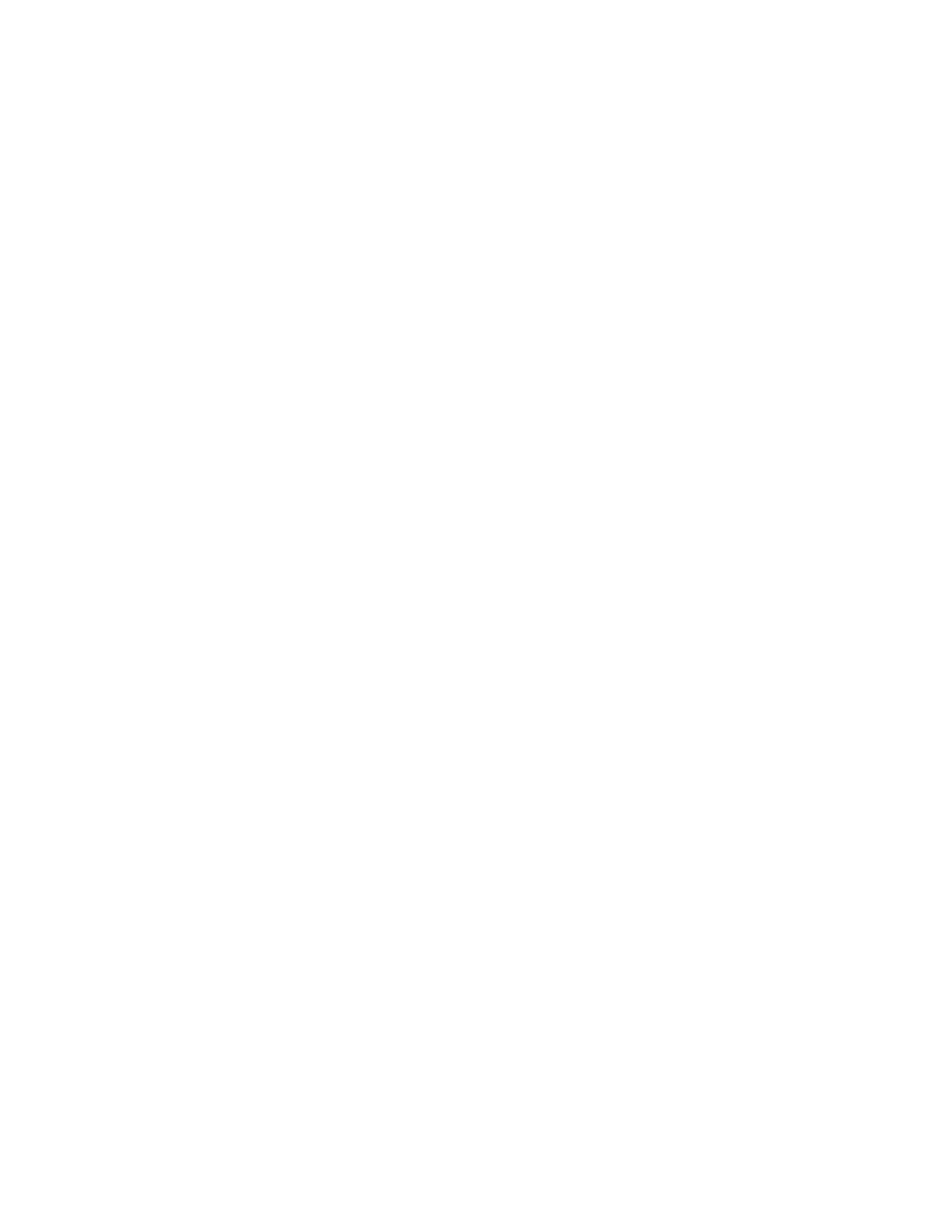
E•A•R 04-01/HP, Gauger and Berger Page - 3
1. INTRODUCTION
Despite the fact that hearing protection devices (HPDs) have been used for occupational hearing
conservation since the early 1950s, widely applied in industry and the military since the early 1970s, and
have been subject to a governmental labeling regulation since 1979 (EPA, 1979), there has never been a
U. S. voluntary consensus standard developed by the American National Standards Institute (ANSI)
specifying how a rating factor should be computed from laboratory derived attenuation data. This is all
the more curious since ANSI has published four standards since 1957 (Z24.22-1957, S3.19-1974, S12.6-
1984, and S12.6-1997) describing how to conduct real-ear attenuation at threshold (REAT)
measurements in a laboratory setting in order to assess the noise reduction that HPDs provide. Because
of the absence of consensus guidance, the development of ratings in the U. S. has been based upon
government reports and regulations (EPA, 1979; Kroes et al., 1975). This contrasts with the Canadian,
European, and Australian/New Zealand experiences in which international or national consensus
standards are the defining documents (CSA Z94.2, ISO 4869-2, SA/SNZ 1270).
Another problem exists with respect to the rating and labeling of HPDs in the U. S., namely the fact that a
number of reports have suggested that the current Noise Reduction Ratings (NRRs) as mandated by the
EPA are an overly optimistic estimator of the actual performance of devices for typical groups of users
(Berger et al., 1998; Berger, Franks, and Lindgren, 1996; Berger and Royster, 1996). The difficulties
have to do with the REAT data from which the ratings are computed, rather than the details of the
computational process itself. However, recent observations suggest that not only should a revised
procedure be specified for the development of the attenuation data, but perhaps a more suitable and
informative rating can be devised as well. In order to redress this problem, ANSI S12/WG11 (Hearing
Protector Attenuation and Performance) has turned its attention to the development of a draft standard to
describe a new HPD rating, designated herein as the Noise Reduction Statistic for use with A weighting
(NRS
A
).
To answer the seemingly straightforward question of the sufficiency of the protection that a hearing
protector can provide one must specify a method of measuring attenuation over a range of suitable
frequencies, include the effects of percentage use time, define the noise exposure of the population or
individual in question, and decide upon a computational method for use of those data (i.e., a rating
scheme). In each of these areas there is a degree of uncertainty with which we must contend. Though
the focus of the current research is the development of a suitable number rating, all four aspects of the
prediction problem will be addressed in the discussion that follows.
1.1 Issues in estimating user protection
Valid estimates of HPD attenuation: To begin, we must of course measure the attenuation of the HPD,
hopefully deriving data representative for the group of users or specific individuals in question, preferably
reflective of their training or skill in use of HPDs. The literature is replete with numerous articles
describing the difficulty of accomplishing this task (Berger et al., 1996, Berger et al., 1998), and American
National Standards Institute Working Group S12/WG11 spent over a decade crafting a new standard
intended to provide improved estimates of field performance (ANSI S12.6-1997). The standard includes
two methods, the latter of which (designated Method B) is the one recommended for the most useful
estimates of field attenuation, hence, Method-B data are the ones primarily used for the analyses in this
paper. Issues regarding the alternative procedure in that standard (Method A experimenter-supervised
fit) are also examined in Sections 2.2 and 3.6. Nevertheless, predictions for specific groups or individuals
based on laboratory attenuation data are rough estimates at best, unless a fit-check approach is
implemented in which the attenuation for the actual users is measured (Berger, 1989; Michael, 1999).